Search
Material selection for bodyworks
- TEAMXLR8
- Oct 29, 2021
- 13 min read
Updated: May 28, 2022
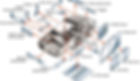
Before we start fabricating any automobile, the first thing is material selection. This will be based on certain important factors such as:
Lightweight
Economical
Sustainability
Safety
Recycling
Why should we consider Lightweight Materials for Automobiles?
Advanced materials are essential for boosting the fuel economy of modern automobiles while maintaining safety and performance. Because it takes less energy to accelerate a lighter object than a heavier one, lightweight materials offer great potential for increasing vehicle efficiency.
A 10% reduction in vehicle weight can result in a 6%-8% fuel economy improvement. Replacing cast iron and traditional steel components with lightweight materials such as high-strength steel, magnesium (Mg) alloys, aluminum (Al) alloys, carbon fiber, and polymer
composites can directly reduce the weight of a vehicle’s body and chassis by up to 50 percent and therefore reduce a vehicle’s fuel consumption. Using lightweight components and high-efficiency engines enabled by advanced materials in one quarter of the U.S. fleet could save more than 5 billion gallons of fuel annually by 2030.
By using lightweight structural materials, cars can carry additional:
Advanced emission control systems
Safety devices
Integrated electronic systems
All without increasing the overall weight of the vehicle.
While any vehicle can use lightweight materials, they are especially important for hybrid electric, plug-in hybrid electric, and electric vehicles. Using lightweight materials in these vehicles can offset the weight of power systems such as batteries and electric motors, improving the efficiency and increasing their all-electric range. Alternatively, the use of lightweight materials could result in needing a smaller and lower cost battery while keeping the all-electric range of plug-in vehicles constant.
How VTO works to improve the Materials:
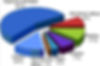
Increasing understanding of the materials themselves through modeling and computational materials science
Improving their material properties
Improving their manufacturing
Developing alloys of advanced materials
In the short term, replacing heavy steel components with materials such as
high-strength.
Steel, aluminum, or glass fiber-reinforced polymer composites can decrease component weight by 10-60 percent. Scientists already understand the properties of these materials and the associated manufacturing processes.

Economical Efficiency:
Uncertainty about future costs is inescapable because of the uncertain rate and direction of future technological progress, as well as uncertainties about the future prices of materials, energy, labor, and capital. Although technological change is certain, its direction, magnitude, and impacts on cost are difficult to predict. For most components, manufacturing costs tend to decrease with increased production volumes and with the accumulation of experience. However, there are no exact methods for predicting future rates of learning by doing or technological progress. Assuming no technological progress or cost reduction via learning will likely overestimate the costs of compliance. On the other hand, overly optimistic assumptions will result in underestimation of costs.
Cost includes three components:
Actual cost of raw materials
Manufacturing value added
The cost to design and test the product
Aluminium and magnesium alloys are certainly more costly and require much energy in the mining and refining processes, than the currently used steel and cast irons. Since cost may be higher, decisions to select light metals must be justified on the basis of improved functionality. Meanwhile the high cost is one of the major obstacles in use of the composite materials.
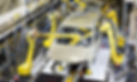
Sustainability:
The importance of some of these factors in the model can be described as follows:

The Resource Depletion Index (RDI):
This index is used to examine the global reserves and the annual consumption rates of certain resources using quantitative measures such as annual mining capacity and total declared global reserves.
Water Pollution Index:
This index represents any chemical or foreign substance contamination into water that is detrimental to human, plant, or animal health by measuring the amount and toxicity of waste water used during the entirety of the vehicle’s lifespan (from the cradle to the grave).
Life Cycle Assessment (LCA):
The LCA is the most-used metric in eco-design. In an LCA, proposed design/material combinations are assessed from energy and emission perspectives over the product’s entire lifecycle.
Recyclability:
Retired or end-of-life vehicles usually undergo one of three scenarios. First, in a landfilling scenario, the whole vehicle or some of its parts are completely disposed in a designated area of a landfill. This strategy is not preferred, as it adds environmental burdens such as contaminations of surface and ground water and loses of the usable land. Second, some vehicle parts (e.g. Body parts and electric components) may be recovered and reused, for
example, by junkyards. Finally, a vehicle’s major parts may be recycled. In this study, we used the percentage recycle fraction (ψ) as the main metric of recyclability.
When a consumer decides not to use a vehicle anymore, there are following options available:
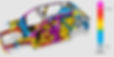
Sell the whole vehicle to another user.
Disassemble the vehicle.
Remanufacture the vehicle.
Recycle the vehicle for materials.
Dispose the vehicle to a landfill.
Durability:
This parameter can either be classified under environmental or economic factors. Nonetheless, durability in sustainability design overlaps all economic, environmental, and societal factors, thus enhancing its versatility. Here, we link durability to environmental factors because of the strong, direct relationship between durability and the replacement or reuse of retired parts. For example, composite-intensive body-in-white (BIW) auto bodies are considered less durable in terms of ultraviolet (UV) resistance than steel BIW auto bodies.
Economic Impact Factors:
These represent the costs associated with each lifecycle phase to provide automakers with a comprehensive financial analysis of a given BIW design.
Societal Factors:
Two metrics are used to quantify societal factors. First, safety is an indirect measure for material properties (i.e., toughness and yield strength). Second, health and wellness are other indirect measures that is governed by:
Noise-vibration-harshness performance (which is controlled by dynamic stiffness of BIW structure and damping capacity of joints and material)
Environmental emissions and ancillary adverse effects (e.g., acid rain, global warming potential, and ozone depletion).
Technical Factors:
This is the fourth pillar of sustainability model for auto bodies. The goal of adding this extra pillar is to highlight the importance of manufacturability (e.g., formability, weldability and joinability, paint ability) and technical requirements within the initial design process (i.e., at the conceptual design stage) by incorporating both material selection and manufacturing process selection. With such criteria, designers can clarify “what if” analyses (e.g., what if material X is used rather than material Y).
Miscellaneous Factors:
Some sustainability factors are qualitative in nature. For example, materials are classified as having high, medium, or low corrosion resistance. Similarly, fatigue resistance and wear resistance can be expressed qualitatively (an example of these scaling is shown in Fig. 8 for scaling strong, wear resistance materials). Moreover, with the absence of a well-established scientific method to quantify societal factors (i.e., safety and health and wellness), scaling may represent a good way to rank candidate materials in terms of performance.
Safety:
Safety can be expressed in many ways; in this study, we define safety from a material selection perspective as the material property that plays a role in determining crashworthiness if a vehicle is involved in any accident including minor crashes.
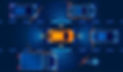
By doing so, safety is assumed to have strong relationship with yield strength and the material toughness.
Similarly, health and wellness can be defined as the material characteristics that interfere with human health and quality of the air.
A simple function of health and wellness should include the amount of emissions released to the environment from cradle to grave (i.e., in all mining and manufacturing processes involved in production and use of the vehicle, and ultimately the recycling or landfilling of the retired parts made from these materials).
Technical factors (i.e. forming, joining, and painting) were also added to the classical sustainability pillars to account for manufacturability of different materials considered in the complex material selection problem.
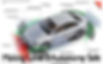
Unfortunately, these technical factors have neither well-established material selection indices nor any material selection charts that designers can use for screening and sorting purposes. For these reasons, we propose using a scale of five classes (very poor, poor, average, good, and very good) to evaluate the performance of materials from the manufacturing perspective.
Materials which are used nowadays:
An automotive body depends on the manufacturer's considerations with the legislation and regulation, and some on the requirements of the customers. Most of the manufacturers prefer featured materials which are lightweight, economic, safety and recyclability.
Steel:
The main elements of selecting material especially for the body is involved in a wide variety of characteristics such as thermal, chemical or mechanical resistance, manufacturing efficiency and durability.

Steel stands as the first choice for manufacturers with all the required characteristics. The improvement or development in the steel industry made the steel stronger, lightweight and stiffer than the earlier.
Steel includes not only vehicle bodies, but also engine, chassis, wheels and many other parts. Iron and steel develop the critical components of structure for the bulk manufacturing of vehicles and are low-cost.
The best reason for using steel as a body structure is its natural capability to absorb the impact energy produced in a crash. Low-carbon sheet steel in the thickness of (0.65–2) mm managed to reduce the overall weight of the car and increase the rigidity of the body.

Types of Steel for Car Bodies:
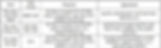
Material scientists are also working towards the development and application of ultra-high strength alloys of the new generation. Some of these are:
Highly-plastic IF-steel;
Steels, which are strengthened during the drying of paint and varnish coating (ВН-steel);
Dual Phase (DP) steels with ferrite-martensitic structure;
Transformation Induced Plasticity (TRIP) steels;
Complex Phase (CP) steels;
Martensitic (Mart) steels;
AHSS (advanced high-strength steels) and UHSS (ultrahigh-strength steels);
High-strength austenitic steels;
High-strength and super-plastic steels of a new generation for lightweight structures (TRIPLEX-steels);
Nano-structured hot-rolled steel NANOHITEN;

Aluminium:
Aluminum is widely used in the automotive industry, in chassis and body structure. Use of aluminum can possibly decrease the weight of the vehicle. Its low weight and high specific energy absorption and precise strength are its most significant characteristics.
Aluminum is resistant to corrosion, but according to its low modulus of flexibility, it cannot substitute steel parts. Hence those parts need to be re-engineered to adopt the same mechanical strength.
Aluminum usage in the automotive industry has vastly grown within less period of time. In the automotive industry, aluminum castings have been used for pistons, cylinder heads, intake manifolds and transmission. In chassis applications, it is used as wheels, for brackets, brake components, suspension, steering components and instrument panels.
Aluminum is used for body structures, finishing and exterior attachments such as crossbeams, doors or bonnets.
Latest improvements showed that 50per cent of the steel is saved for the body in white by the substitution of steel by aluminum. This can result in up to 20-30 per cent reduction in the total weight of the vehicle.

There has always been a lot of competition between materials, so to choose material we should talk about the different aspects of steel and aluminium.
The Battle of the Bodies - Steel vs Aluminium:
On the periodic table, aluminium is about half as dense as iron. So, it is lighter than steel. Automakers like Tesla and Ford choosing aluminum bodies in order to translate the weight savings to the battery or payload capacity.
In other hand the tensile strength of steel is up to 2,000MPa (290,000psi), which is about four times stronger than the strongest aluminum alloys available today.
This tensile strength difference means that aluminum parts need to increase thickness to meet safety standards, while advanced high-strength steel (AHSS) can be made thinner, thereby reducing weight. Granted, these thicker aluminum parts may still come out lighter than their steel counterparts.
You may get lower mass with aluminum, but it’s a relatively small difference, and you pay for that mass reduction because of the higher cost of the material.
As a rule of thumb, automakers largely ballpark the cost of aluminum materials at two to three times higher per kilogram than steel.
Vehicle safety- Aluminium vs High strength Steel:
Aluminum-bodied vehicles are safer than their steel counterparts thanks to better energy absorption, larger crush zones that fold more predictably and larger overall size. But the counter point is it’s not the material that matters so much as how you design with that material.
Again, one can say the counterpoint that every aluminum-bodied vehicle on the road has a five-star safety rating. But all are newer, higher-end vehicles. Luxury vehicles can afford the extra engineering to get that rating.
So, we can conclude that aluminum can be as safe as steel—but at higher cost.
Sustainability of Aluminium vs Steel:
While aluminum can be easily recycled through advanced processes, steel has the advantage of being the most recycled material in the world.
The recycling process of steel is simpler, too. Its ferrous property allows for easy scrapyard sorting, and all alloys of steel can be melted together and remixed to produce any alloy of steel.
Aluminium, on the other hand, is costlier to recycle, requiring the different grades to be separated before melting to preserve the grades.
So, as per requirements we will use aluminum or steel.
Magnesium
Magnesium is another light-weight metal that is growing increasingly next to aluminum in automotive engineering.
It is 33% lighter than aluminum and 75% lighter than steel elements. Magnesium components have many mechanical disadvantages that need a unique design for utilization to automotive products.
Magnesium has lower tensile strength, fatigue strength, and creep strength compared to Aluminum.
The modulus and hardness of magnesium alloys are lower than aluminum, while the thermal expansion coefficient is greater.
As it has low mechanical strength, pure magnesium cannot be used, must be alloyed with other components.
The most common alloying components for room temperature applications is Mg-Al-Zn group, which include aluminum, manganese, and zinc.
Modern Polymer and Composite Materials:
The polymer composites in automotive applications today are glass fiber-reinforced thermoset polymers used mostly in non-structural parts of the vehicle especially for low- and mid-volume cars and trucks. Fiber reinforced thermoplastics and, especially, carbon fiber reinforced thermosets show great potential, the latter having twice the weight reduction potential of glass fiber reinforced thermoset polymers.
Fiber-reinforced thermoplastics share the advantageous properties of polymer matrix composites and are also recyclable, have indefinite shelf life, and feasible for automated, high volume processing with a potential for rapid and low-cost fabrication. The cost is the single most major barrier for the limited application of polymer composites in automobiles today.
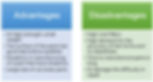
Future of materials used in bodyworks:
Here we will talk about some materials which can be the future of automobile industry. They are light weight, sustainable, safe, and recyclable. Long research works are going on them.
Carbon fibre composite material:
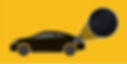
Weight is a vehicle’s worst enemy. It slows down fast cars, and makes any vehicle less fuel efficient. To lop off the extra flab, automakers use lightweight materials wherever possible.
One of the best-known is carbon fiber. It’s used extensively in racing and in supercars, and it’s starting to trickle down into more mainstream consumer vehicles – including GMC’s all-new 2019 Sierra, where it’s used in the truck bed, and BMW’s electric i3, where the body’s made from it. But it’s still relatively rare in the auto world. That’s because it’s expensive, simply because of what’s involved in making it.
Still, for certain applications, it can be worth the cost. The strongest carbon fiber can be ten times stronger than steel, and eight times stronger than aluminum, but at considerably less weight. And unlike metal, when it’s made with the right resin, carbon fiber doesn’t corrode.
In 1981, McLaren produced its MP4/1, the first Formula 1 car made using carbon fiber. It was controversial, until two things happened that year. The lightweight car won the British Grand Prix, and then it crashed at high speed at the Monza Grand Prix. It might have been a disaster in an aluminum car, but thanks to the stronger material, the driver walked away unharmed. Carbon fiber had arrived.
Automakers aren’t the only clients. Carbon fiber is also widely used in the aerospace industry; almost half of Boeing’s 787 Dreamliner is made of composite material, including carbon fiber. That has been a boon for the auto industry, since it spreads the cost of development: Lamborghini and Boeing share a carbon fiber research facility.
Manufacturing:
Every carbon fiber manufacturer uses slightly different proprietary methods, but overall, the processes are similar.

It starts as strands of fibers. Most are made of a thermoplastic material called polyacrylonitrile, or PAN, which is also used to make wool-like acrylic fibers for clothes or upholstery. Rayon, petroleum distillate, or other chemical compounds can also be used.

The strands are heated, without oxygen and at very high temperatures. Because of the lack of oxygen, they don’t burn, but carbonize into chains of interlocked carbon atoms.
The fibers are then oxidized with liquid or gas, so the resin will stick to them, and coated with a protective material, such as epoxy or polyester. Then the fibers are spun together, just like yarn.

Where they go from there depends on how they’re going to be used. They can be woven into fabric sheets, which give the finished part the familiar cross-hatch pattern that most associate with carbon fiber.
The strands must be blended with resin, where both combine to form a carbon fiber reinforced plastic (CFRP) composite that’s stronger than either material alone.

When using carbon fiber sheets, a mould is made for the part. The fabric sheets are impregnated with an epoxy resin and pressed into the mould.
The mould goes into a bag and all the air is vacuumed out, and then it’s baked in an oven for several hours. It’s a very time-consuming process, requiring either a skilled worker or an expensive robotic machine to layer the fabric in the mould.
A combination of chopped carbon fiber and resin can be pressed into a mould to make parts that are too complex for layering.

Depending on the resin used, it may still to go into an oven for several hours to be cured. It’s not quite as strong as the woven mat, but it’s cheaper, and it’s sturdy and light compared with metal.
Chopped carbon fiber and resin can also be sprayed onto a backing, such as foam, fiberglass or plywood, to create parts made from the resulting composite sandwich.
To make tubes, more commonly used in aerospace than automotive, resin-impregnated yarn is wrapped in overlapping layers around a mandrel. Some companies are also working on 3D printers using CFRP to build up parts.
This could be used to create even more complex designs, such as honeycomb, which in itself adds strength and reduces weight when made from conventional plastics or resins.
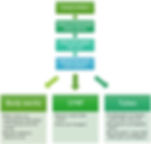
Disadvantages:
Though carbon fiber has so many advantages, there are some disadvantages also.
Beyond its price, carbon fiber does have some disadvantages. It can be repaired, but it requires special techniques, and repairs can’t be done on unpainted parts because the fix is visible.
Cutting shapes for parts out of woven carbon fiber sheets produces expensive waste.
The finished carbon fiber parts are also difficult to recycle. The fibers are the valuable part, and the resin has to be dissolved or burned off around them. The current methods also result in recycled fibers that aren’t as strong as new ones.
Carbon fiber may be firmly established as an automotive material, especially at the higher end of the scale, but it’s still constantly being studied and refined.
Futuristic material with multi-functional capability:
The newly produced material is stiff like metal and also flexible to absorb vibrations.
The new material in question can withstand vigorous vibrations and has superior sound dampening qualities.
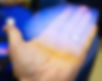
The researchers used layers of 3D woven technical textile composites that have selected unbounded fibers.
This arrangement of layers will enable the middle layers of the textile to move while the outside layers stay rigid. This is how the new material maintains high rigidity while having dampening abilities.
This combination of properties is very rare in common materials found around us and rightfully so because as rigidity increases, it results in less movement of internal particles, resulting in low damping abilities.
The opposite can be said for dampening materials because as the packing between the internal layers become loose, more will be its dampening ability.
This is why we see metals having high rigidity with a low dampening ability and a material like foam being less rigid but having high dampening ability.
This new invention by the researchers is undoubtedly impressive and can have a major impact on the materials used in the manufacturing of many products that require both these properties.
Think about replacing the standard body panel materials like aluminum and steel with this new material. Cars and even airplanes will become more silent in their operation.
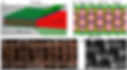